Content
- A brief history of hydrogen
- Why is carbon important for H2?
- How to make H2 and what powers H2 storage?
- What about money and safety?… Transport and Steel?
- What is “White” hydrogen… and the other colors of the rainbow?
- Summary
Links and Resources (here you can find all links and sources mentioned in the article)
On 20 July 1969, Neil Armstrong was the first man ever to step foot on the Moon. His “space vehicle” was powered by liquid hydrogen LH2 [1].
Hydrogen is a wonder molecule, the lightest known on Earth… powerful enough to fly us to the moon!
Since then, a few things have changed. Coal, oil, and gas – providing us with over 80% of all energy we use – have been doomed by the UN Chief as the masters of all disasters, causing unprecedented “boiling” of our beloved planet Earth. Someone (I don’t know who) came up with the glorious idea to make that wonder molecule hydrogen, the same molecule which propelled humanity into space 1, form a key building block for the future of our energy systems. The idea is that hydrogen will be able to largely replace oil, coal, and gas for power, thermal, and product production purposes, by leaving nothing but clean water H2O after its “combustion”… what could be more desirable?
Most, if not all, energy enthusiasts understand by now that in regards to the “energy transition” hydrogen is NOT A SOURCE of energy, but “only” AN ENERGY CARRIER. An energy carrier means that it will be “produced” from energy first in order to “carry” energy from point A to B or over a longer period of time, thus hydrogen “costs” energy.
In the press and scientific reports, it seems people either love or hate H2, either “believe” it is the future or “express” that it will lead to energy suicide. Let’s dig deeper into this hot topic, the molecule H2 and try to drown out some of the noise.
Note: today’s blog refers to our book “The Unpopular Truth… about Electricity and the Future of Energy”, recently updated to the 2024 edition, so be sure to watch the video!
[1] Liquid H2 is used as a rocket fuel because 1) it provides more thrust per unit of fuel => it combusts at very high temperatures , 2) it is very light, high energy density per kg, 3) at -250°C it can be compressed tightly, despite it being expensive and difficult to handle.
- A brief history of hydrogen
In 1766 the philosopher, chemist, and physicist Henry Cavendish is said to have discovered the “inflammable air” hydrogen that formed water on combustion. Scientists had been producing hydrogen for years before it was recognized as an element. Written records indicate that Robert Boyle produced hydrogen gas as early as 1671 while experimenting with iron and acids [2].
But then the 1937 Hindenburg disaster occured. A spark is said to have ignited leaking hydrogen, setting fire and destroying the Zeppelin, filled with H2. This was where the public became aware for the first time of the dangers of hydrogen [4].
Still, after centuries of study we estimate that 90% of the visible universe is composed of this powerful hydrogen.
Hydrogen is the same “raw” fuel that most stars ‘burn’ to produce energy. Fusion, the process that stars use, is now being studied as a possible power source for use here on Earth. The sun is expected to shine another 5+ billion years because that is the estimate how long its supply of hydrogen will last [2].
By the way, liquid hydrogen is important in cryogenics and in the study of superconductivity, as its melting point is only 20 degrees above absolute zero (about -250°C).
On Earth, hydrogen occurs mainly in combination with oxygen in water, but it is also present in organic matter such as living plants, petroleum, coal, etc. (Figure 2).
For decades, hydrogen has been produced and consumed primarily for industrial purposes. Total global hydrogen demand is estimated at ~120 Mt. It is mostly used in oil refining and ammonia NH3 production through a process called “Haber Process” (NH3 is crucial for fertilizers/food production).
Figure 1: Sources of Hydrogen
Secondary uses include blending with other gases for the production of steel and methanol. H2 is also added to fats and oils, such as peanut oil, through a process called hydrogenation. Liquid hydrogen is used in the study of superconductors and, when combined with liquid oxygen, makes that excellent rocket fuel.
It is estimated that today’s hydrogen originates 99,96% from fossil fuels, natural gas (~60%), coal (~20%), and oil (~20%), see Figure 1
For example, the IEA’s [18] estimate for “net-zero” is based on one of several unrealistic assumptions that 530 Mt of “green hydrogen” are required annually by 2050. This would take over 27.000 TWh every year (equal to todays’ almost entire global power generation, assuming 50 kWh/kg power required for electrolysis). If this hydrogen were used only for storage and repowering, then only 5.500-9.500 of this 27.000 TWh would be usable to the end consumer.
The hydrogen atom is a principal energy carrier in many chemical fuels because of its reactivity in accepting and releasing the energy in its chemical bonds with other atoms. Furthermore, it is the lightest molecule, giving it a very high gravimetric energy density (energy per kilogram). On the other hand, H2 has a very low volumetric energy density (energy per liter, see Figure 3).
A low volumetric energy density means that H2 cannot be economically transported over large distances, nor can it be economically stored over the longer term, it is so thin that it literally “seeps through everything”, even steel.
Today, H2 is considered or hoped to be the solution to long-duration energy storage. H2 is supposed to overcome one of the principal nature driven problems of wind and solar: intermittency. As a result, H2 will attract 100s of billions of US dollars in funding in the coming years.
Figure 2: Fuels in a Thermodynamic System C-H2-O2; Conversion of Carbohydrate to Coal, Methane and Liquid Hydrocarbons. Source: Wolf 2021, from our book www.unpopular-truth.com (downloadable image available there)
2. Why is carbon important for H2?
Carbon is another lightweight element that is an excellent energy carrier and fuel component. Coal, which is primarily carbon, combusts in oxygen with an energetic output.
When it comes to hydrogen, carbon is a chemical miracle worker. Combined with hydrogen, carbon forms highly versatile and energetic hydrocarbon gaseous and liquid fuels (i.e. natural gas, gasoline, diesel).
Hydrocarbons can be easily stored and transported at ambient temperature and pressure conditions. Higher carbon ratios yield solids, and lower ratios yield gases (Figure 2). Carbon also performs the magic of packing hydrogen atoms much more closely together. This explains why gasoline and octane contain over 60% more hydrogen atoms per m3 than pure liquid hydrogen.
Because carbon adds its own significant energy to the mix, gasoline has ~3,5x higher volumetric energy density (kWh per liter) than liquid hydrogen, and ~7x higher density than compressed H2, as envisioned for H2-powered cars (Figure 3).
Only a few solids have a higher volumetric energy density than liquid gasoline, which explains its wide-spread use for transportation.
Thus, carbon transforms hydrogen, a low-density, explosive gas that liquefies at -250°C, into a room-temperature hydrocarbon liquid (e.g., gasoline, diesel or other petroleum products (CxHxOx). These hydrocarbons have over three times the energy density of hydrogen alone.
In addition to being so thin, literally penetrating everything, hydrogen has a wide range of flammable concentrations in air and lower ignition energy than gasoline or natural gas, which means it can ignite far more easily. Hydrogen is thus very dangerous to be handled without special equipment and training… Keep out of reach of children
“If we didn’t have carbon, we would have to invent it as the ideal tool for handling hydrogen” (Kiefer 2013, p117, [3]).
H2 is 8x lighter than methane, to compress H2 to 200 bar ~9% of its energy content is lost vs. ~2,5% when pressurizing methane
H2 is a synthetic energy carrier: High-grade energy is required to produce, compress, liquefy, transport, transfer or store H2 (in most cases this energy could be distributed directly to the end user from wind or solar)
Figure 3: Volumetric energy densities of hydrogen, gasoline, and natural gas. | Source: Schernikau based on Bossel Eliasson 2006, Bossel 2009, Wikipedia on Energy Densities | (1) Diesel has slightly higher volumetric energy density than gasoline, at 38 MJ/l. More detailed notes available at graphs at the website
3. How to make H2 and what powers H2 storage?
We explored what today’s “hydrogen economy” is used for and where that hydrogen comes from, aka fossil fuels. We also explored the importance of carbon for making the most abundant molecule hydrogen “useful” at ambient pressure and temperatures. Next, we explore the “energy transition’s” dream to use hydrogen for long duration energy storage (LDES) purposes in order to overcome the intermittency problem of wind and solar.
Making hydrogen for storage purposes, requires energy, lots and lots of energy. The current estimates are around 50-60 kWh of electricity per 1 kg of hydrogen [6], translating to over 27.000 TWh for 500+ million tons H2 envisioned for “net-zero” according to IEA [18]. But wide ranges of 40 to 80 kWh/kg are also reported. 55 kWh seems to be a reasonable number, 50 kWh too optimistic, but in the end, it doesn’t really matter, The number remains high.
How much is a lot? Well, let’s say we want to “make” the 120 mln tons of today’s annual hydrogen consumption using electrolysis, how much electricity would we need? More than 6.000 TWh is the answer, about as much power as the entire US and 3x Germany consume every year (Figure 1).
Producing hydrogen for storage “costs” or “loses” 65-80% of the input energy for electrolysis, compression, storage, transportation, and repowering. That means 65 to 80% of the electricity you use to “make” hydrogen for storage is “wasted”. Since energy is never lost, this “wasted” energy is released in the form of low value, high entropy heat, into our atmosphere, logically warming our biosphere around us.
Oh, hydrogen combusts at 2.130 °C and the flame is hardly visible. This creates further safety and even emissions issues because it also reacts so easily with nitrogen in the air.
Let’s consider, if so much energy is “wasted” then you would logically rather try to use the electricity directly, meaning you would first use any electricity available from wind or solar to charge your Tesla first. Only then would you use any left over, unused electricity to produce hydrogen for storage. You would logically never consider to to use gas, coal (with or without CCS), nuclear, hydro or geothermal, all perfectly fine and usable forms of electricity without unnecessary waste, to make hydrogen for storage
You would logically ONLY use unutilized, excess wind and solar power to produce hydrogen for storage, everything else would be energy and economic “lunacy”.
In 2023 the world generated a total of 3.900 TWh of intermittent wind and solar power. It takes over 6.000 TWh of consistent (not intermittent) power for electrolysis just to replace the existing 120 mln tons of H2 the world consumes today (not for storage). Electrolysis efficiency reduces drastically with an intermittent power source, see Figure 5.
Remember that we are installing TWs of wind and solar to “save the climate” by reducing the greenhouse gas emissions of our energy systems. GHG emission reduction is supposed to limit future “global warming” which is supposed to reduce extreme weather events and “halt” sea-level rise. Shouldn’t hydrogen from wind and solar, then reduce CO2 emissions?
Well, let’s have a look. The IPCC AR6 [7] states that solar has a lifecycle greenhouse gas footprint of 9-250g/kWh (including its albedo effect 2), that is a very wide range. We are finalizing a new peer-reviewed paper on this subject, showing that even assuming most of the unrealistic assumptions made by the IPCC’s research – this number is likely to be at the upper end.
Hydrogen produced from electrolysis and then repowered, from the current German grid (almost 40% wind and solar), would emit twice as much as an “unabated” coal fired power station (Figure 3). If you transport H2 in some sort of liquid form, it will get significantly worse because of boil off and liquefaction.
Using a conservative 200 g/kWh lifecycle CO2eq footprint for solar on average, with a 100% solar grid, “green” hydrogen would equal about the same relative CO2eq emissions as a coal fired power station (950 g CO2/kWh)
All of this is only true if you incorrectly assume there is zero CO2 footprint associated with electrolysis, compression, storage, repowering equipment and related processes.
[2] Albedo relates to the fraction of incident radiation that is absorbed by dark surfaces and therefore heats the surrounding biosphere.
4. What about money and safety?… Transport and Steel?
About the money: in this article, I don’t spend too much time on money and costs for H2 but want to give you some of the key numbers. When you understand the “energy waste” that occurs in producing hydrogen for storage as explained above, you also understand that it must be expensive. How expensive depends on location and method. Here some snippets
- Various institutions claim that green hydrogen in the US costs 5 USD/kg, and may drop to lower than 2 USD/kg [9]
- Interestingly, the cost of producing hydrogen from coal with CCUS (Carbon Capture Utilization and Storage) would be about three times less expensive than producing low-carbon hydrogen through water electrolysis [20]
- Electrolyzer prices have held steady at around $2.000/kW and are expected to remain at that level at least through 2025 [10]
- Even at $500/kW electrolyzer cost and using the strong UK wind conditions (amongst strongest in the world), H2 cost would still remain above 5 USD/kg (Figure 4). I would challenge even that number.
- Over 33% of 2050 electricity generation (over 27.000 TWh of 65.000 to 80.000 TWh) could be required to produce hydrogen for storage in “net-zero” scenarios
- The world’s largest 260 MW Kuqa hydrogen project in China by Sinopec finds it “difficult” to work with intermittent wind and solar input. “Firstly, the reliability of renewable energy sources can be erratic, with fluctuations in wind and solar power affecting the consistency of hydrogen production.” (BNEF/Hydrogen insight [15])
By 2030, the expected investment in “green” hydrogen is projected to be substantial. McKinsey estimates that around US$ 320 billion will be invested in hydrogen projects by 2030. Hydrogen Council suggests that achieving the 2050 net-zero carbon emissions target requires tripling investments in hydrogen projects to US$ 700 billion by 2030.
The IEA [18] estimates “green” hydrogen investments of over US$ 1 Trillion by 2050 to reach “Net-Zero”. This is indeed a lot of money to be played with, made profits from… and in the end wasted. Imagine what good be done for eradicating poverty, improving education & health, better our environment and general living conditions.
Europe admitted that it needs to import hydrogen, so Germany’s chancelor visited African countries to see if those power starved nations may not use some of their scarce energy for Germany. The idea is that African nations produce hydrogen with their electricity that should then be “exported” to Germany, with those heavy energy losses, to make Germany “green” (sources here). Will energy become the new colonialism?
The safety concerns of wide-spread hydrogen use, have been discussed at length in various reports and was illustrated by the Hindenburg disaster in 1937.
Hydrogen is used and handled safely in massive quantities in industry daily. However, moving H2 to higher pressures than we use in industry, and doing this for commercial or consumer, rather than for industrial purposes, is going to come at some cost, ultimately involving loss of life. Explosions in the US, Europe, Asia, and Australia tell countless stories. Fan et al. from Columbia titled a recent study “Hydrogen Leakages: a potential risk for the hydrogen economy” [16].
By the way, apparently leaked hydrogen has a global warming potential over 20 years GWP20 37x higher than CO2 and 12x higher at GWP100. This at least according to Sand et al. 2023 who calculated these but also point out large uncertainties [16]. Hydrogen is not directly a GHG, but its chemical reactions change the abundances of the GHGs methane, ozone, and stratospheric water vapor, as well as aerosols.
For transport: when it comes to hydrogen for transport via fuel cells, one has to understand that similar challenges exist as with H2 storage. Gasoline or even battery-powered vehicles are far more energy-efficient than hydrogen powered vehicles. On LinkedIn Michael Sura explains this in detail also in relation to steel. Similar holds true for liquid hydrogen-based aircrafts. “Hydrogen as a fuel is a square wheel”.
NASA can tell all the stories about transporting and storing liquid Hydrogen LH2. If NASA “loses” 45% of its liquid hydrogen for space travel, then I am certain standard global operators have trouble doing better [NASA 13].
For steel: The idea is to use hydrogen for the chemical removal (reduction) of oxygen from iron ore in its solid form. This technology attracts a lot of attention because it enables the production of so-called “green” steel. Let’s for now disregard that such processes require much higher quality iron ore as an input, which is scarce and expensive. If hydrogen was “green” or “zero CO2”, which it is not, as explained in the previous section, then “green” steel is to compete with “fossil” steel in price.
The “green” hydrogen used for “green” steel production would have to cost less than 1 EUR/kg. At a price of 5 EUR/kg H2, the “none-green” steel from H2 would cost about 2 times more than currently.
5. What is “White” hydrogen… and the other colors of the rainbow?
Maybe it is also time to talk about some other colors.
White Hydrogen – natural occurring H2, extracted from Earth
Blue Hydrogen – H2 produced from natural gas using steam reforming, with carbon capture and storage, CCS
Green Hydrogen – H2 produced from wind, solar, or hydro power using electrolysis, assumed to be zero CO2, which is incorrect
Pink Hydrogen – H2 produced from nuclear using electrolysis
Grey or black Hydrogen – H2 produced from gas (grey) or coal (black) using steam reforming or gasification, no CCS
The most interesting is clearly naturally occurring White Hydrogen. Because if we had naturally sourced low-cost hydrogen available for energy use, clearly a significant portion of our energy problems could be solved.
Natural “white” hydrogen has been discovered in oceanic spreading centers, transform faults, passive margins, and other settings. The primary sources of such hydrogen include alterations in Fe(II)-containing rocks, the radiolysis of water, magma, and the reaction of water- and silica-containing rocks during the mechanical fracturing. Currently, natural “white” hydrogen exploration is in its infancy. I highly recommend Lu et al 2023: The Origin and Occurrence of Natural Hydrogen for more details [5].
Various “white” hydrogen start-ups started already to raise significant funds [6]. I fully support the search for white hydrogen. Such a source would be a true game changer. My concern is not about finding a long-term solution to our energy problems which may include fusion and “white” hydrogen. I am completely sure that we will solve this long-term energy “problem”.
My concern is for the economic and environmental mistakes made in current energy policy, focusing on wind, solar and derived “green” hydrogen for storage or transportation purposes.
Let me say a word or two about blue, pink, grey, and green hydrogen.
- Blue hydrogen for storage is such a waste… why not use the gas directly?
- Pink hydrogen for storage is such a waste… why not use nuclear directly?
- Grey hydrogen for storage is such a waste… why not use the gas or coal directly?
- Green hydrogen for storage is such a waste… and will never sustainably solve the intermittency problem of wind and solar. Sustainable = economically and environmentally
To illustrate, SHELL recently scrapped its goal to produce up to 450.000 tonnes/year of “blue hydrogen” in Norway by the year 2030. Subsequently, Equinor scrapped the plan for a hydrogen pipeline from Norway to Germany [8].
6. Summary
Hydrogen produced using wind and solar power with electrolysis is supposed to overcome one of the principal nature driven problems of wind and solar, which is intermittency, attracting hundreds of billions of US dollars in funding in the coming years.
The fallacy of considering hydrogen as a “sustainable” part of any future “energy transition” has to do with the fact that 65 to 80% of the electricity used to “make” hydrogen for storage is “wasted”. The fact that “green” hydrogen does not really exist is illustrated by this simple back-of-the-envelope calculations.
- Using conservative 200 g/kWh lifecycle CO2eq footprint for solar on average, with a 100% solar grid, “green” hydrogen would equal about the same relative CO2eq emissions as a coal fired power station (950 g CO2/kWh)
- Current “net-zero” pathways as envisioned by the IEA would require almost as much electricity in 2050 – just for H2 production – as the entire world consumes in 2024.
It seems that concerns around hydrogen are slowly being recognized. For instance, in its latest 2024 Renewable Report the IEA said that hydrogen-dedicated “renewable” energy capacity is expected to grow 35% lower than forecasted a year earlier, but still by 45GW [17].
Or even more recently in its Oct 2024 “IEA Renewable Report”, the organization stated: “Hydrogen remains a negligible driver for new renewable capacity growth” [19, p7]
Let me ask you a final question: If hydrogen is not workable for long duration energy storage … doesn’t that make the entire “transition” to wind and solar power not workable? Because as far as I understand, the entire “net zero transition” is based on “zero” CO2 wind and solar plus some “zero CO2” short and long duration energy storage!
As Michael Sura often says, “to produce hydrogen for storage or transportation purposes is a square wheel”. I add, “and the single biggest waste of scarce and expensive electricity”.
For more details on hydrogen, I recommend Prof. Furfari’s book “The Hydrogen Illusion” [10]
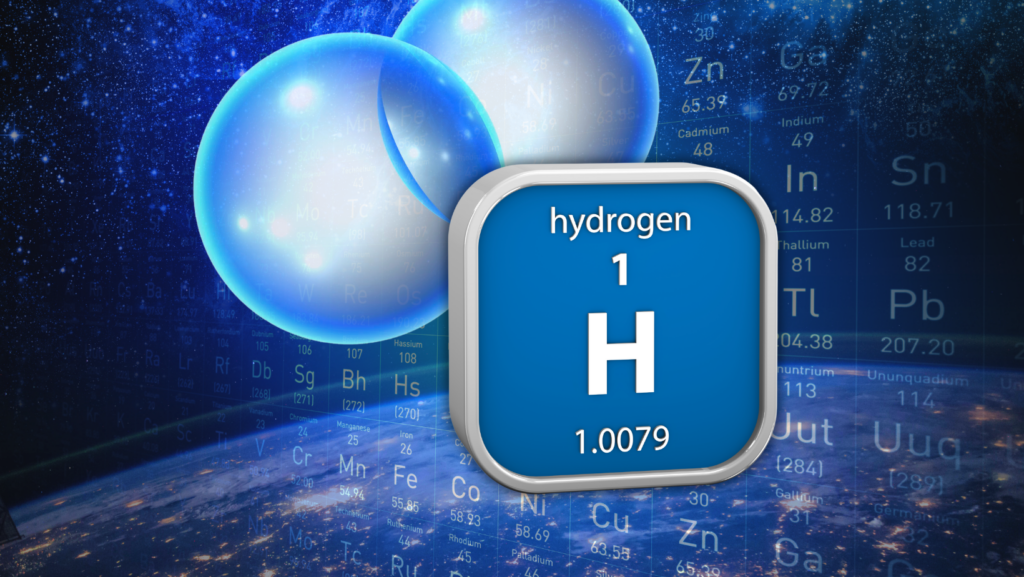
Links and Resources
[1] NASA on Liquid Hydrogen https://www.nasa.gov/missions/artemis/innovative-liquid-hydrogen-storage-to-support-space-launch-system. and https://www1.grc.nasa.gov/wp-content/uploads/Centaur-First-Liquid-Hydrogen
[3] Kiefer, Capt. Ike 2013, Twenty-First Century Snake Oil, Feb 2013, see page 6 in Chapter 3.3 on hydrogen and page 15 in Chapter 5 on eROI, https://uwaterloo.ca/complexity-innovation/sites/ca.complexity-innovation/files/uploads/files/kiefer-snake-oil31.pdf
[4] Wikipeida on 1937 Hindenburg disaster https://en.wikipedia.org/wiki/Hindenburg_disaster
[5] Lu et al 2023: The Origin and Occurrence of Natural Hydrogen.” Energies 16, no. 5 (January 2023): 2400. https://doi.org/10.3390/en16052400.
[6] Bill Gates and Jeff Bezos Back White Hydrogen Startup, OilPrice.com, https://oilprice.com/Energy/Energy-General/Bill-Gates-and-Jeff-Bezos-Back-White-Hydrogen-Startup.html.
[7] IPCC AR6, 2021, https://www.ipcc.ch/assessment-report/ar6/
[8] on Shell and Equinor in Norway, Sep 2024, https://www.linkedin.com/posts/michael-sura-9a47511bb_hopium-hydrogen-squarewheel-activity-7246437030652448769-bBrf, https://www.linkedin.com/posts/michael-sura-9a47511bb_hydrogen-hopium-squarewheel-activity-7244677476218454016-BYKi/?utm_source=share&utm_medium=member_ios
[9] Net-Zero Steel in Building and Construction: The Way Forward | McKinsey, April 2022.
[10] BCG: Global Trade in Hydrogen Will Miss 2030 Targets, Nov 2023. https://www.bcg.com/publications/2023/accelerating-global-hydrogen-trade.
[11] IEA: World Energy Outlook 2023 – Analysis, Oct 2023. https://www.iea.org/reports/world-energy-outlook-2023.
[12] Global Energy Perspective 2024 | McKinsey. Sep 2024. https://www.mckinsey.com/industries/energy-and-materials/our-insights/global-energy-perspective.
[13] NASA on Liquid Hydrogen Storage Losses, 2011.
[14] Hydrogen: Missing Link to a Livable Climate: How Hydrogen-Enabled Synthetic Fuels Can Help Deliver the Paris Goals,” September 2020. https://www.lucidcatalyst.com/hydrogen-report
[15] World’s Largest Green Hydrogen Project ‘Has Major Problems Due to Its Chinese Electrolysers’, December 2023 and Sep 2024. https://www.hydrogeninsight.com/production/exclusive-worlds-largest-green-hydrogen-project-has-major-problems-due-to-its-chinese-electrolysers-bnef/2-1-1566679 and https://energynews.biz/green-hydrogen-project-falls-short-in-china/
[17] Reuters: IEA Lowers Renewables Forecast for Clean Hydrogen Reduced.” February 2024. https://www.reuters.com/business/energy/iea-lowers-renewables-forecast-clean-hydrogen-2024-02-01/.
[18] IEA’s 2022 updated roadmap to NetZero, https://www.iea.org/reports/world-energy-outlook-2022/an-updated-roadmap-to-net-zero-emissions-by-2050
[19] IEA: Renewables 2024 – Analysis.” IEA, Oct 2024, https://www.iea.org/reports/renewables-2024.
[20] based on IEA and other data, summarized by FutureCoal https://www.futurecoal.org/coal-facts/